Why is chemistry important?
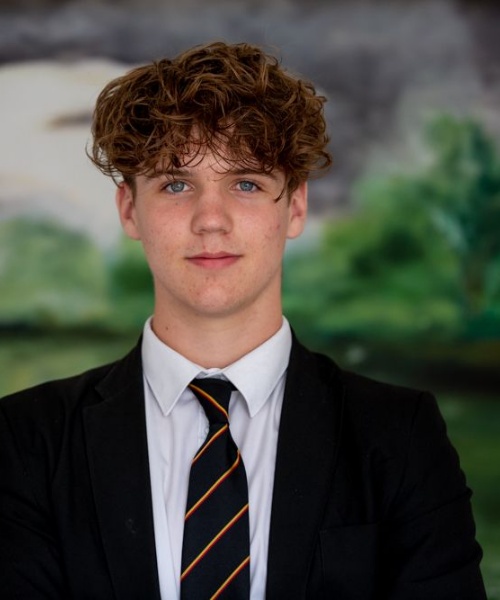
Why is chemistry so integral to our lives? Everything we see is an application of chemistry, argues Benjy (Yr12), who won the Peterhouse Kelvin Science Essay Competition with this essay.
Why is chemistry important?
While all the sciences are undoubtedly incredibly important fields of research in the modern day, chemistry, the study of electrons and reactions, holds a vital role in tying all the various disciplines that make up science together. Biology would have no conception if it weren’t for chemistry eloquently unravelling the secrets of DNA, proteins, and the inner workings of the cell. Physics and engineering would have a hard time formulating and applying new materials for hyper-fast vehicles and research equipment. Chemistry plays a crucial role in integrating all these various disciplines and is therefore why it is so important for us as a species and for the future of the planet.
It is good to always keep in mind that everything we use is likely to have travelled through the hands of a chemical researcher at some point in its history.
We will start with a brief section on how chemistry is present in, and critical to life, one part of the discipline which is very close to home for all living beings.
There is much more chemistry that goes into railways and transport which we don’t see. Given the speed of high-speed trains, it would be understandable if one were to miss seeing one, even despite consuming copious amounts of vitamin A - It would be gone in the blink of an eye.
“Eating carrots lets you see in the dark”. I’m sure you’ve heard this phrase once before by your parents, sometime early in your childhood perhaps. But is there any truth to this saying? Well, more than one would expect. Carrots contain a highly important dietary molecule called beta-Carotene, which the body metabolizes into another molecule called Retinol, or more commonly, Vitamin A. Vitamin A is converted into Retinal and is present predominantly in the rods in our eyes, the microstructures that allow us to detect light. Coupled with a protein called Rhodopsin, when a photon collides with it, cis-retinal undergoes a “Cis-trans photoisomerization” reaction, prompting a complex chain of reactions, and finishing with a signal being sent down a nerve to the brain, allowing us to see. These are some applications of chemistry in the body. (Kiser, et al., 2013)
On the topic of signals, hormones, and the endocrine system play another crucial role in transmitting them throughout an organism; they too are powered by chemistry. Hormones are proteins or molecules that react with different organs and cells and often cause long-term responses, with a major exception being epinephrine-Adrenaline. One of the most well-known hormones is Dopamine. It is produced in the brain as well as the adrenal glands and acts between the synaptic gaps of neurons in the reward system of the brain. Its production is generally stimulated by things that our primal bodies would have required thousands of years ago for survival, such as food and social interactions. (Konkel, 2022) Some hormones are proteins, not molecules, which are fundamental polymers, necessary for all variation in living beings. Proteins are made of monomers called amino acids. This is due to the presence of amine and carboxylic acid functional groups in the molecule. There are only 20 different amino acids, and they handle all variations between species in the world.
But how does an organism sequence these proteins? One of the most well-known polymers, DNA, which stands for Deoxyribonucleic Acid, is imperative in this process. It is a double-helix polymer that contains many monomers called nucleotides. Nucleotides have a common sugar-phosphate backbone where they bond to each other, and then a base which differentiates them into Adenine, Guanine, Cytosine, Thymine, or Uracil. Groups of 3 of these monomers are called Codons and they are responsible for “coding” the sequence of amino acids in a protein chain. Hydrogen bonds are also present between the helix, holding them together to ensure the robustness of the genetic code. Now, these biological polymers are integral to life, but none of this would be possible without chemical reactions and the seemingly simple bonds present between the atoms.
Taking a small step away from biochemistry, organic chemistry studies all things carbon. This branch is incredibly interesting to look into, but also is an indispensable research field that fundamentally fuels the entire global economy. If it weren’t for this discipline, almost all products we use daily would simply not exist; it can’t be stressed enough how important organic chemistry is in the modern day, so at least a few applications of it should be mentioned.
Crude oil is the primary raw material organic chemists work with to synthesize cosmetics, textiles and fuels. From the rig, oil is shipped or pumped to chemical refineries across the globe, where the mixture of hydrocarbons is heated into a vapour and condensed at different temperatures, called fractions. Some fractions like petroleum and kerosene are used every day, fuelling our aircraft, cars, trucks, and vehicles. Other fractions like naphtha are further refined and reacted which go on to create more unexpected things. One of the most common reactions naphtha undergoes is cracking, where alkane chains are broken down into alkenes and other products. Alkenes, like Ethene, have many more uses which we use to synthesize almost all other organic chemicals. Most notably, they can be polymerized to create plastics and fabrics. Polyethylene, the polymer made from Ethene, is used in many applications such as in bottles, containers, and components in electronics. The computer you are using right now probably contains a generous amount of this polymer and the bottles we buy from the shop are made of PET (Polyethylene terephthalate). Other common plastics include ABS, PVC, and Acrylic. Many modern consumer products would be impossible without these polymers, and I think this highlights just how important chemistry is in this part of our everyday lives.
Synthetic fabrics are also just as interesting when it comes to organic chemistry and product synthesis. Many clothes are made of polyesters, which are polymers made up of repeating ester monomers formed from crude oil constituents. I can guarantee you that at least one, if not all the items of clothing you are wearing right now are made up of synthetic fabrics or mixes such as polycottons. In terms of chemistry, the monomers for the fabric are polymerized and then drawn out into threads, much like the way cotton is. They are then spun into a thin twine which is weaved into cloths, ready to stitch into high fashion dresses, or all the way down to a simple T-shirt for daily use. Scientists and researchers are always looking for ways to improve these polymers and create new, stronger textiles. A high-performance material called Kevlar was discovered and researched by a woman called Stephanie Kwolek in 1964. It was developed by her and released to the public in 1971 after years of developing a better process to deal with this tough fabric. Kevlar is generally used for military applications in bulletproof vests, where strength and durability are integral to keeping a soldier alive and well in a warzone. (Bristol, School of Chemistry, 2002)
As Kevlar is generally found in military situations, it would be a good time to talk about the various applications that chemistry has in combat and materials research.
On many stealth fighters, RAMs (radio-absorbing materials) are often coated on the bottom side of the aircraft to absorb or dampen radio waves sent out from enemy radar. One of the most common coatings contains ferrite spheres which are suspended in a paint or polymer. These absorb EM waves and discharge the energy as heat which soon after is conducted by the body of the aircraft, making it less detectable by the enemy. Other materials work in a similar way, dispelling the absorbed EM waves as heat into the surroundings. (Mouritz, 2012) Other developments in this field of research are going towards making stronger, lighter, and longer-lasting components for fighter jets. Carbon fibre, although incredibly strong, is very expensive, so researchers are looking into ways to create new composites which can compete with this versatile material while being less expensive in the process.
Light materials like this will come all the more important as we move to the future. With electric and more fascinatingly hydrogen-powered vehicles on the road, the demand will shift to manufacturing cars with efficiency even more in mind. Hydrogen-powered cars will likely become a major part of a greener and more sustainable future. By moving to this type of power, chemistry will be necessary for strategizing the transportation, storage, and production of Hydrogen, as well as for the creation of fuel cells which utilize multiple inorganic and physical chemistry principles to operate. With these vehicles, no CO2 emissions are produced and only water is dispelled as a by-product. There are no harmful greenhouse gasses produced by these energy converters so they could be the first completely renewable fuel source for cars in the next decade or so. (Fuel Cell & Hydrogen Energy Association, n.d.)
Hydrogen’s slightly heavier neighbour Helium, although in relatively short supply, also has some very practical uses in transportation, other than floating houses in Pixar movies. Currently being developed are train systems that run on Maglev “tracks”, essentially floating the carriages above the surface. These are incredibly fast and eliminate all contact friction which is present with conventional wheels. To enable these cabins to levitate, superconducting electromagnets made of a niobium-titanium alloy, are used which require an incredibly low temperature of 4°K. (Central Japan Railway Company, n.d.) In order to get down to these temperatures, liquid Helium and Nitrogen are used, as well as a thick insulating wall of polystyrene to keep out the heat. Chemical engineers had to innovate a way to condense Helium from a gas into a liquid using high-pressure condensers. This technology is now actually being used in Shanghai, China. One of their maglevs can travel at a top speed of 431 km/h, allowing you to cross the city in a matter of minutes. This would not have been possible if it weren’t for the work of these chemical engineers and many other disciplines of science joining together, to create this feat of modern engineering.
And, on the topic of train transportation, chemistry becomes vitally important when it comes to traditional rail. Train-tracks are made of a complex alloy of steel where a crystalline structure is formed in the metal called “Pearlite”. It is strong and stiff and is coated with zinc at the bottom to help with corrosion using sacrificial protection. Most rails are bolted to each other to allow for easy maintenance and for thermal expansion, however, high-speed rail requires tracks to be welded together. Yet again, like in many industries, a handy chemical reaction called the thermite reaction is utilized. Iron Oxide and Aluminium powder are mixed and ignited in a mould. This highly exothermic reaction cascades, forming a product of molten iron which melts into the place around the track, essentially fusing the rails together. This creates an uninterrupted, single smooth line which is perfect for high-speed applications. (Chapman, 2022) There is much more chemistry that goes into railways and transport which we don’t see every day, but it is good to always keep in mind that everything that we use is likely to have travelled through the hands of a chemical researcher at some point in its history.
Given the speed of some of these high-speed trains, it would be understandable if one were to miss seeing one, even despite consuming copious amounts of vitamin A - It would be gone in the blink of an eye.
So why is chemistry so integral to our lives? I would answer by asking you to look around the environment you are in. Everything we see is an application of chemistry in one form or another, from deep into outer space, to common daily items we use without even thinking. What makes chemistry even more important is the fact that it will shape everything that happens in the future regarding technology, life, and maybe even the fate of humanity. It forms such an important part of our lives today and has since the start of life itself, and that is why chemistry is so important.
Benjy T (Upper Sixth)
References
Bristol, School of Chemistry, 2002. History of Kevlar. [Online]
Available at: http://www.chm.bris.ac.uk/webprojects2002/edwards/history.htm#:~:text=Kevlar%20was%20first%20synthesized%20in,Delaware%20in%20the%20United%20States.&text=Kwolek%20was%20born%20in%20New%20Kensington%2C%20Pennsylvania%20in%201923.
[Accessed 23 April 2023].
Central Japan Railway Company, n.d. About Maglev. [Online]
Available at: https://scmaglev.jr-central-global.com/about/#:~:text=In%20the%20Superconducting%20Maglev%20system,452%20degrees%20Fahrenheit%20below%20zero.
[Accessed 23 April 2023].
Chapman, K., 2022. How chemistry keeps trains on track. [Online]
Available at: https://edu.rsc.org/feature/how-chemistry-keeps-trains-on-track/4016560.article#:~:text='%20In%20rails%2C%20the%20most%20common,the%20cables%20in%20suspension%20bridges.
[Accessed 23 April 2023].
Fuel Cell & Hydrogen Energy Association, n.d. Fuel Cell Basics. [Online]
Available at: https://www.fchea.org/fuelcells
[Accessed 23 April 2023].
Kiser, P., Golczak, M. & Palczewski, K., 2013. Chemistry of the Retinoid (Visual) Cycle. [Online]
Available at: https://www.ncbi.nlm.nih.gov/pmc/articles/PMC3858459/
[Accessed 23 April 2023].
Konkel, L., 2022. What Is Dopamine?. [Online]
Available at: https://www.everydayhealth.com/dopamine/
[Accessed 23 April 2023].
Mouritz, A., ed., 2012. Radar Absorbing Materials. In: Introduction to Aerospace Materials. s.l.:Woodhead Publishing.
Olguín, H. J., Guzmán, . D. C., Mejía, G. B. & García, E. H., 2015. The Role of Dopamine and Its Dysfunction as a Consequence of Oxidative Stress. [Online]
Available at: https://www.ncbi.nlm.nih.gov/pmc/articles/PMC4684895/
[Accessed 23 April 2023].